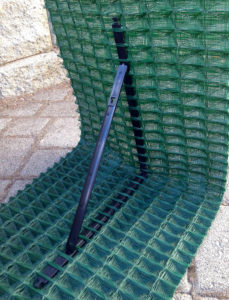
New patented technology is available to construct geosynthetic wrap-face vegetated (GWFV) retaining walls and reinforced soil slopes using a pyramidal-woven, high-performance turf reinforcement mat (HPTRM) in conjunction with fiber-composite internal braces. This wrap-face system eliminates the need for temporary external bracing (formwork) or for facing elements comprised of metallic wire-frame units, which traditionally have served as a bulkhead to allow mechanical compaction of backfill soil directly behind the face. In many cases, on-site soil can be used for infill, provided it contains little organic detritus and does not include fine-grained elastic soil. Vegetation can be seeded or planted during construction or post-construction, depending on the specific application and site conditions. Straight and curved alignments are possible, and the GWFV system is especially well-suited to stream-bank, wetland, littoral and coastal applications; for landslide remediation; and as wing walls for construction of a geosynthetic reinforced soil–integrated bridge system (GRS–IBS).
Geosynthetic wrap-face system
The internally braced wrap-face system is comprised entirely of geosynthetic components (Miller 2016), starting with a woven polypropylene HPTRM, which has suitable porosity, longevity and wide-width tensile strength. The bracing components are made of a high-strength, fiber-composite material consisting of a nylon and fiberglass blend, and they are woven through the pyramidal projections of the HPTRM (Figure 1) and assembled to stand up the fabric at the face to allow soil filling and compaction for each 1-foot-high (0.3-m) lift (Figure 2). Only small, hand-operated vibratory compactors should be used directly adjacent to the face.
The rolled HPTRM is 8.5 feet (2.5 m) wide, providing a typical wrap layer with 4 feet (1.2 m) along the bottom, 1 foot (0.3 m) for the near-vertical face and 3.5 feet (1.1 m) folded back over the compacted soil infill. This layered GWFV system produces a reinforced soil mass (Figures 3a and 3b), which is designed to resist lateral earth pressures acting on typical structures up to 8 feet (2.4 m) high and provides excellent erosion protection as vegetation seeded within the infill soil emerges and becomes established. The semi-open weave of the fabric is also conducive to the establishment of vegetation by hydroseeding after the wrap-face wall or slope has been constructed.
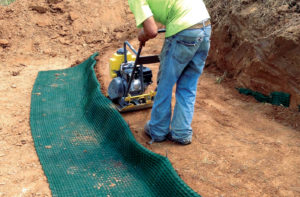
To construct GWFV structures exceeding 8 feet (2.4 m) tall, an additional geosynthetic element is added to the system; that is, longer reinforcement is required within the backfill zone to serve as the primary reinforcement in the traditional sense for reinforced earth structures. This is achieved by sandwiching geogrids or strong woven geotextiles in between successive lifts and extending them back as far as needed to meet geotechnical design criteria. Soil is spread in thin layers in the interface zone to ensure that geosynthetic sheets are not in direct contact with one another. Internal stability is achieved using a woven geosynthetic or geogrid with sufficient wide-width tensile strength and by vertically spacing the longer reinforcements at no more than 2 feet (0.6 m). Typically, the vertical spacing may be reduced to 1 foot (0.3 m) in the lower portion of the backfill zone to resist the greater lateral earth pressures at this depth.
The HPTRM, by design, enhances grass establishment and root-system reinforcement. It also provides sufficient tensile strength and UV resistance for longevity of such wrap-face systems. Additionally, the fabric loft (thickness of 0.4 inches [1 cm] prior to being compressed in the backfill zone) allows the embedded sheets to provide lateral drainage to help transfer free groundwater out to the face, thus eliminating the need for granular chimney drains near the face, common to modular-block retaining walls.
The GWFV walls or reinforced soil slopes (RSS) must be constructed directly on native soil or approved structural fill. Subgrade preparation involves an excavator with a smooth-blade bucket removing topsoil and organic detritus to reach native, undisturbed soil to provide a smooth, shallow trench for placing the initial wrap-face lift. If the foundation soil comprises clay or elastic silt, which are moisture-sensitive soils, then a compacted-gravel leveling pad must be placed, along with a pipe drain system, to remove any excess groundwater. If the subgrade trench requires grade changes to conform with natural topography, then the base of the wrap-face structure must step properly to horizontally align the wrap lifts (Figure 4). Construction begins at the lowest elevation, then progresses upward in steps. If a foundation drain pipe is used, then it must have a proper outlet for water disposal or conveyance at these low points.
Geotechnical design considerations
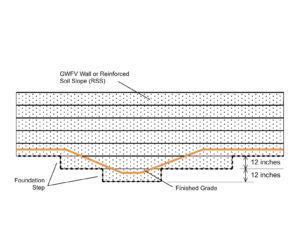
Geotechnical engineering practice distinguishes between retaining wall design and slope design depending on the planned face angle of the reinforced-earth structure. RSS design methods apply for slopes up to 70˚ (1H:2.75V), whereas for face angles steeper than that, the structures are considered reinforced-earth walls (Berg et al. 2009). The steepest recommended face angle for the new GWFV system is 76˚ (1H:4V).
For wall design, the focus is on evaluating the stability against the following potential failure modes (Berg et al. 2009): 1) base sliding; 2) overturning (i.e., rotational tipping of the wall); 3) exceeding foundation bearing capacity; 4) internal failure of the geogrid (i.e., rupture or pullout); and 5) large-scale global slope failure. These stability evaluations lead to design specifications for the required minimum length of the geogrid reinforcement zone behind the face, the vertical spacing of the geogrids and the minimum wide-width tensile strength of the geogrids, which typically is expressed in terms of LTDS (long-term design strength) as pounds per linear foot width (lb/ft).
For RSS design (Berg et al. 2009, Rimoldi 2016), the focus is on large-scale global slope stability analysis (Abramson et al. 2002), which considers potential failure surfaces external to the reinforced soil zone as well as those passing through the reinforced zone (i.e., internal compound stability). Depending on face angle, backslope angle, soil strength and geogrid strength, the critical failure path may or may not pass through the reinforced zone. Final geotechnical design recommendations prescribe geogrid lengths, vertical spacings and minimum LTDS. Often to achieve the desired global stability, a tighter vertical spacing and greater length of geogrids are needed in the lower portion of the reinforced zone (Figure 5).
Versatile applications
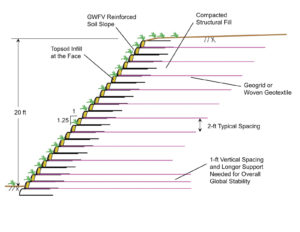
Potential applications for GWFV structures include everything from small landscaping enhancements for residential and commercial properties to large-scale earthwork grading needed for transportation, land-development and landfill projects. Most land-development projects today require a stormwater management component that involves installation of stormwater detention facilities, which may include ponds or basins that temporarily hold runoff water from intense precipitation events, then gradually release that water in a controlled fashion. If these basins can be constructed deeper and with steeper perimeters (banks), then more usable land is preserved in the vicinity.
Figure 6 illustrates a GWFV wall sloped at 72° (1H:3V) serving as the perimeter of a detention basin. The infill soil at the face should be topsoil, which can be incorporated as a separate part of the infill zone by using a 15-inch-high (38-cm) batter board placed vertically in the infill zone as the soil is placed. This divider typically is a strip of plywood placed parallel to the wall face and about 6 to 8 inches (15 to 20 cm) behind the HPTRM face. Topsoil is placed on the face side of the divider, and approved structural fill is placed on the backside away from the face to the prescribed height. Then, the plywood divider is pulled out, and the lift is mechanically compacted. This process results in excellent growth media at the face where it is needed and structural fill soil (organic and detritus free) within the reinforced zone.
During infilling of each wrap lift, grass seeds or stolons can be added to the soil directly behind the HPTRM at the face; rhizomatous grasses are preferred, because they spread laterally by surface or shallow runners to fill in open areas and produce a solid ground cover. If desired, additional seeding can be achieved post-construction by hydroseeding the completed wall.
By adding geosynthetic extensions (typically, woven geotextiles) to serve as primary reinforcement in the backfill zone, GWFV walls provide an economical, green option for wing walls on GRS–IBS projects. Abutment slopes/walls directly beneath the bridge comprise modular block walls or gabion walls to protect against stream scour, and because lack of sunlight and moisture often preclude a vegetated alternative. However, such hardscape facings easily can transition to a GWFV option for the wing walls.
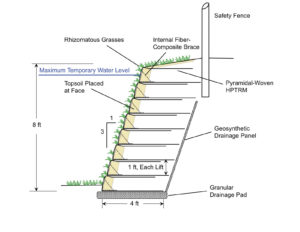
Such geosynthetic reinforced soil (GRS) structures are suitable for small bridge abutments and approaches because of the following assumptions (Adams et al. 2012): 1) a GRS mass is not supported externally, and, therefore, the facing system is not considered a structural element in design; 2) lateral earth pressure at the face of a GRS mass (i.e., thrust) is not significant, eliminating connection failure as a possible limit state; and 3) the facing elements of a GRS mass are frictionally connected to the geosynthetic reinforcement.
Besides seeded grass or herbs, other vegetation such as bare-root stock of vines (creepers), small shrubs or cuttings from meristem-rich plants can be inserted between wrap layers during construction with their leafy tops protruding out of the face (Figure 7). If needed and available, temporary limited irrigation can be used to help new plantings get established, taking care that excessive water is not applied to the wall system.
When landslides or localized excessive erosion damage a roadway prism or embankment, reconstruction of the affected slope often can be achieved using geosynthetic reinforced earth systems. In many cases a gabion or
wire-form-faced retaining wall with reinforced soil backfill is an economical choice that can be constructed in a timely manner. A GWFV wall or RSS also can be a good option that provides a green sustainable slope repair, as illustrated in Figure 8. The face geometry may be adjusted to offer various slope angles as well as planting benches for insertion of

Figure 7 An example of a GWFV wall at 76˚ (1H:4V) used as a wing wall for GRS–IBS construction.
potted plants or bare-root stock. Small holes cut into the HPTRM will not significantly affect its overall performance, and the fabric highly resists unraveling because its joints are tightly knotted in the pyramidal weave. If the slope repair is in a riparian area along a stream, then rock riprap may be used at the slope toe to resist scour and protect the lower wrap lifts until the brush cuttings take root and begin growing and spreading.
If a steep slope is required for new construction or for a slope-stabilization project involving soil nails or ground anchors, then a GWFV wall can serve as a vegetated “veneer” covering for the soil-nail slope (Figure 9) rather than a more typical
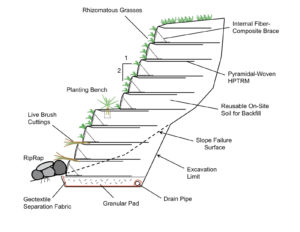
hardscape, such as shotcrete. Such a project would proceed with top-down construction to install the soil nails (or ground anchors) to the desired elevation, where a narrow working bench is established. The GWFV wall then is constructed from the working bench upward, with the back end of 4-foot-long (1.2-m) flaps being attached to the all-thread nails/anchors using plates and nuts. This construction option is also suitable for any site having limited space for excavation and placement of an extended geogrid-reinforced backfill zone.
Percussion-driven pins or ground anchors can be used on the flap tails in between the soil-nail rows. If the working face comprises a more rocklike material, then the pins will be grouted all-thread dowels or percussion-driven friction bolts. In many cases, on-site soil may be acceptable for the wrap-face infill, and, thus, native soil excavated to install the nails/anchors and form the working bench can be reused for wall backfill.
Often, for stabilization of riverbanks, lake shorelines, coastal and littoral areas (including tidal lagoons and estuaries), the lower wrap lifts that are planned to be below average water level are filled with coarse granular backfill, such as ¾-inch (2-cm) gravel, to allow free movement of water. Wrapped lifts placed above the water level then transition to finer-grained infill
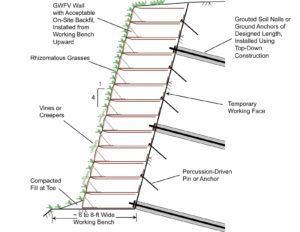
soil to support wetland vegetation (Figure 10). Because the GWFV system consists solely of geosynthetic components, which are environmentally inert and do not corrode, it is ideal for wet installations and especially for saltwater applications using brackish-tolerant plant species. It offers a valuable green alternative for projects focused on coastal restoration and protection.
Conclusions
This new GWFV wall and slope system is easily constructible and requires no special tools or equipment. Often, the on-site soil can be used as backfill in the reinforced zone, provided it is free of organic detritus and is not a fine-grained, moisture-sensitive soil (i.e., clay or elastic silt). The use of lower-quality on-site soil in the backfill often is an economic advantage over importing better-quality structural fill. Also, even if significant settlement does occur after construction, the GWFV system is much more forgiving to such deformations than more rigid facing materials, such as modular concrete blocks, wire-form panels or gabions.
For remote, heavily wooded or steep project areas with difficult equipment access, the ability to easily transport or carry geosynthetic materials to the site is an advantage over heavier modular blocks or metal panels. Remote sites along lakeshores, rivers, estuaries and coastlines could be accessed by boat, readily transporting materials and workers to the projects. Once the leveling pad or stepped foundation is prepared, the HPTRM is rapidly rolled out along the wall line, ready for brace installation and soil infilling. If the planned GWFV wall is no higher than 8 feet (2.4 m), then over-excavation is limited, and the HPTRM serves both as the geosynthetic soil reinforcement and the facing.
Depending on the specific application, seeds or stolons can be placed with the infill soil or bare-root stock inserted in between successive wrap lifts during construction,
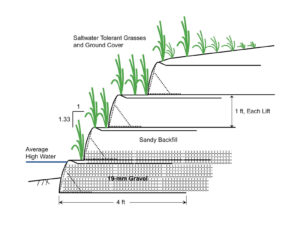
or potted plants and hydroseeding can be added post-construction. A landscape professional or plant scientist should be consulted to evaluate and select the plant species most likely to become established and sustainable along the wrap-face structure. Temporary light irrigation may be prescribed for the first few months after the project is constructed.
Finally, site investigation and characterization of geotechnical soil properties are required to provide reasonable confidence in the wall or RSS design results. Construction oversight and monitoring is strongly recommended to confirm the site conditions concur with those assumed in the design analysis and to verify that construction practices follow the engineering design and specifications.
Stanley M. Miller, Ph.D., P.E., is a professor emeritus in the Department of Civil Engineering at the University of Idaho in Moscow, Idaho, and a senior engineer with STRATA Inc., a geotechnical consulting firm in Boise, Idaho. His areas of expertise include applied rock engineering, geosynthetic reinforced earth structures and slope stabilization, and he is a licensed professional engineer in six western states.
All photographs and illustrations courtesy of the author.
Abramson, L. E., Lee, T. S., Sharma, S., and Boyce, G. M. (2002). Slope stability and stabilization methods, 2nd ed. Wiley, New York.
Adams, M., et al. (2012). “Geosynthetic reinforced soil integrated bridge system interim implementation guide.” FHWA-HRT-11-026, USDOT, Federal Highway Administration, Washington, D.C.
Berg, R. R., Christopher, B. R., and Samtani, N. C. (2009). “Design of mechanically stabilized earth walls and reinforced soil slopes.” FHWA-NHI-10-024 and FHWA-NHI-10-025, USDOT, Federal Highway Administration, Washington, D.C.
Miller, S. M. (2016). “Combining geosynthetics to construct steep vegetated slopes and walls.” Land and Water, 60(6), 31–38.
Rimoldi, P. (2016). “Geotextiles used in reinforcing walls, berms, and slopes,” in Geotextiles: From design to applications, ed. by R. M. Koerner. Elsevier, Amsterdam, The Netherlands.
It is good material on account of enviroment depledation
Yes, this GWFV wall system could be used with conventional shotcrete facing. However, the cost of this face wrap (HPTRM intended for vegetation growth) is much greater than traditional woven geotextiles that are used for wrap-walls. If shotcrete facing is required for the project, then woven high-strength geotextiles will be a more economical option.
GWFV, CAN IT BE USED WITH SHOTCRETE FOR CONVENTIONAL STRUCTURES ??
Yes, this GWFV wall system could be used with conventional shotcrete facing. However, the cost of this face wrap (HPTRM intended for vegetation growth) is much greater than traditional woven geotextiles that are used for wrap-walls. If shotcrete facing is required for the project, then woven high-strength geotextiles will be a more economical option.
Great Product! meets all the different code requirements, improves the aesthetics of a any site and hopefully long term stability and cost effective.
Good day,
I have saw the article about GWFV walls , new system, and I’m very interested in it..
Our company is based in Italy, working in centre Europe
can we have more details or info about it ?
Ciao Sr. Fusato,
I apologize for the delay in replying. I just now saw your posting on the website. I am familiar somewhat with geotechnical work in Italy, having conducted a sabbatical leave at the Politecnico di Torino in 2007.
This new GWFV system is available from Propex Global; additional information is available at the link below. Also, I can send additional information and project photographs if you are interested.
http://propexglobal.com/GeoSolutions/Product-Tour/Pyrawall
Best regards, Stan Miller