Introduction
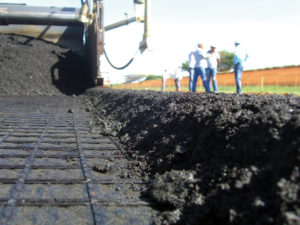
The geosynthetic products most commonly used in roadway systems include geotextiles (woven and nonwoven) and geogrids (biaxial and multiaxial), although erosion-control products, geocells, geonets (or geocomposite drainage products), and geomembranes have also been incorporated in a number of projects. These various types of geosynthetics can be used to fulfill one or more specific functions in a variety of roadway applications. For example, geosynthetics have been in use since the 1970s in applications aimed at improving the performance of unpaved roads on soft subgrade soils. Beginning in the 1980s, geosynthetics were used in applications that focused on minimizing reflective cracking in asphalt overlays as well as on improving the performance of base aggregate layers.
The terminology used in the technical literature to describe the various applications of geosynthetics in roadway systems as well as the functions of these geosynthetics when incorporated into roadway applications has not been consistent. This is understandable because the mechanisms that lead to roadway improvement in each application are complex and often intertwined. Consequently, a framework is presented in this article that is expected to minimize inconsistencies regarding the terminology used when designing roadways using geosynthetics.
While strongly based on the terminology currently used for geosynthetic design (e.g., Koerner 2012), the refined framework proposed herein follows two key premises: (1) Different geosynthetic functions unequivocally correspond to different geosynthetic properties, and
(2) Geosynthetic applications correspond to the different roadway projects that are implemented to achieve specific design purposes. Each geosynthetic application may involve a single geosynthetic function or a combination of such functions, fulfilled by mechanical or hydraulic mechanisms, which ultimately enhance the roadway performance.
Functions and applications
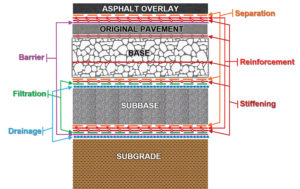
Figure 1 shows a paved road section with the location of possible geosynthetic layers and the various functions that these geosynthetics can fulfill. These
functions include:
Separation–The geosynthetic, placed between two dissimilar materials, maintains the integrity and functionality of the two materials. It may also involve providing long-term stress relief. Key design properties to perform this function include those used to characterize the survivability of the geosynthetic during installation.
Filtration–The geosynthetic allows liquid flow across its plane, while retaining fine particles on its upstream side. Key design properties to fulfill this function include the geosynthetic permittivity (cross-plane hydraulic conductivity per unit thickness) and measures of the geosynthetic pore-size distribution (e.g., apparent opening size).
Reinforcement–The geosynthetic develops tensile forces intended to maintain or improve the stability of the soil-geosynthetic composite. A key design property to carry out this function is the geosynthetic tensile strength.
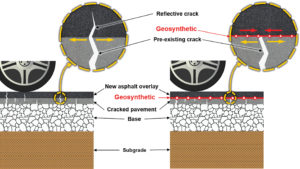
(a) roadway designed without geosynthetics, (b) roadway designed with geosynthetics.
Stiffening–The geosynthetic develops tensile forces intended to control the deformations in the soil-geosynthetic composite. Key design properties to accomplish this function include those used to quantify the increased stiffness resulting from the soil-geosynthetic interaction.
Drainage–The geosynthetic allows liquid (or gas) flow within the plane of its structure. A key design property to quantify this function is the geosynthetic transmissivity (in-plane hydraulic conductivity integrated over thickness).
While comparatively less common in roadway applications, additional geosynthetic functions include:
6. Hydraulic/gas barrier–The geosynthetic minimizes the cross-plane flow, providing containment of liquids or gases. Key design properties to fulfill this function include those used to characterize the long-term durability of the geosynthetic material.
7. Protection–The geosynthetic provides a cushion above or below other material (e.g., a geomembrane) to minimize damage during placement of the overlying materials. Key design properties to quantify this function include those used to characterize the puncture resistance of the geosynthetic.
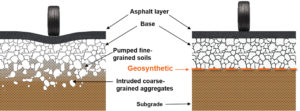
geosynthetics, (b) roadway designed with geosynthetics.
Six of the seven functions listed above have traditionally been reported in the technical literature (e.g., Zornberg and Christopher 2007, Koerner 2012). However, an additional function—stiffening—is considered in this article. This addition is deemed appropriate to make a clear distinction on whether the geosynthetic acts as a mechanical inclusion to develop tensile forces for the purpose of improving the system stability or of controlling its deformations. While both functions result in mechanical improvements, the properties required to fulfill them are distinctively different.
One or more of the seven aforementioned geosynthetic functions are used to enhance the roadway performance in the following five roadway applications: (1) mitigation of reflective cracking in asphalt overlays; (2) separation; (3) stabilization of road bases; (4) stabilization of road soft subgrades; and (5) lateral drainage. This list is limited to applications of geosynthetics within the actual roadway section. Consequently, it does not include
transportation applications aimed at enhancing the roadway performance but that involve components beyond the roadway section. Such applications, including trench drains, erosion control elements, and surface water management features, are discussed by Holtz et al. (1997, 2008) and Zornberg and Thompson (2010).
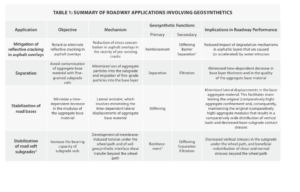
Table 1 identifies a total of five roadway applications involving geosynthetics. For each of the five roadway applications, the table identifies the design objectives, relevant mechanisms, the primary and secondary geosynthetic function(s) involved in road application, and the implications in roadway performance.
Following on this general overview of the different geosynthetic functions involved in roadway applications, each one of these applications are described next. Specifically, an overview of the two initial applications listed in Table 1
(i.e., mitigation of reflective cracking in asphalt overlays and separation) are provided in Part 1 of this article. Part 2
of this article addresses the remaining three applications listed in Table 1 (i.e., stabilization of road bases, stabilization of road soft subgrades, and lateral drainage).
Mitigation of reflective cracking in asphalt overlays
The prevention of reflective cracking in asphalt overlays was one of the earliest applications involving geosynthetics in paved roads.
Reflective cracks often develop in new flexible pavement overlays directly above the location of preexisting cracks within the old paved road. Reflective cracking may be triggered by bending and/or shear stresses induced by repeated traffic loads as well as by tensile stresses caused by thermal variations (Button and Lytton 2003).
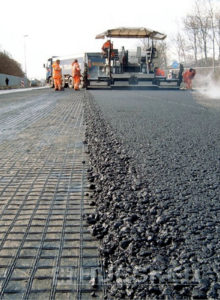
Figure 2a shows the development of stresses resulting from lateral movements induced by flexing and shearing of the asphalt overlay at the location directly below the traffic load. Such stresses may end up causing a reflective crack that propagates through the new pavement overlay, making it susceptible to moisture intrusion and early failure facilitated by. Geosynthetics have been used to mitigate the early development of reflective cracks through one or a combination of functions (Perkins et al. 2010). These functions include reinforcement, stiffening, barrier and separation (or stress relief).
Accordingly, the geosynthetic can act:
By developing tensile forces in the vicinity of the crack tip, thereby reducing strains in the bituminous material to prevent the triggering of new cracks. This reinforcement function has been achieved using polymeric, steel or glass grids.
By providing a layer that allows horizontal displacements so that potentially large movements can develop without failure in the vicinity of preexisting cracks. This mechanism has been referred to as stress-relief interlayer, often involving bitumen-impregnated nonwoven geotextiles and can be characterized as a controlled debonding.
By providing a hydraulic barrier function to waterproof the underlying roadway layers, even after the reappearance of a crack in the road surface. This mechanism has also involved the use of bitumen-impregnated nonwoven geotextiles.
The use of a geosynthetic to fulfill a reinforcement function at the interface between the surface of an old pavement surface and a new overlay is shown in Figure 2b. The figure illustrates the geosynthetic tensile forces that can halt the progression of reflective cracking. Montestruque (2002) conducted laboratory tests on reinforced and unreinforced asphalt concrete beams to investigate the use of geosynthetic reinforcement in pavement overlays. Polymeric geogrids and a nonwoven geotextile were used as reinforcements. Results indicated better performance of the geogrid-reinforced specimens as compared to the geotextile-reinforced and unreinforced specimens. More recently, Correia and Zornberg (2016) reported that the use of geosynthetics as reinforcement in asphaltic layers can not only mitigate the development of reflective cracks, but can also increase the structural capacity of paved roads.
Figure 3 illustrates the use of geosynthetics in a project involving a polymeric geogrid placed between the existing asphalt layer and the new asphalt overlay to mitigate the potential development of reflective cracks into the overlay.
Separation
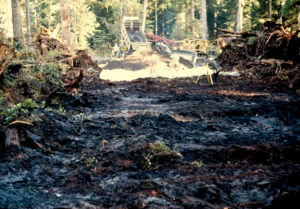
Geosynthetics were first used in roadway applications solely to fulfill the function of separation. In this application, a geosynthetic is placed between two layers of soil with different particle-size distributions. Indeed, a major cause of failure of roadways constructed over soft foundations is the contamination of aggregate base material with the underlying soft subgrade soil (Figure 4a).
Contamination may occur due to: (1) penetration of the aggregate into the weak subgrade after localized bearing capacity failure under wheel-induced stresses, and (2) intrusion of the fine-grained soils into the aggregate because of pumping or subgrade weakening due to excess pore water pressures. Subgrade contamination results in inadequate structural support, which often leads to premature failure of the roadway. A geosynthetic placed between the aggregate and the subgrade can act as an effective separator by preventing mixing of the subgrade and base aggregate materials.
Even a small amount of fines contaminating a granular layer can negatively affect its structural response, including a reduced shear strength, decreased hydraulic conductivity, and increased frost susceptibility. Ultimately, a mix involving base aggregate material contaminated with fine-grained soils would essentially behave as the fine-grained soil itself. Consequently, the contamination effectively leads to a reduced base layer thickness and, ultimately, to a decreased road life.
Use of a geosynthetic separator is comparatively inexpensive and may result in significant cost savings over the design life of the roadway (Figure 4b). Among the different types of geosynthetics, geotextiles have generally been used to achieve the function of separation. Design methodologies for the use of geosynthetics in separation applications are provided by Holtz et al. (1997, 2008) and Koerner (2012).
Figure 5 shows the use in the 1970s of a geotextile as separator between the visibly poor subgrade material and the newly placed base aggregate.
References
ASTM (1995). ASTM Standards on Geosynthetics, sponsored by ASTM Committee D35 on Geosynthetics, Fourth Edition, 178 p.
Button, J.W. and Lytton, R.L. (2003). “Guidelines for Using Geosynthetics with HMA Overlays to Reduce Reflective Cracking.” Report 1777-P2, Project Number 0-1777, Texas Department of Transportation, Austin, Texas.
Christopher, B.R. (2014). “Cost Savings by Using Geosynthetics in the Construction of Civil Works Projects,” Proceedings of the 10th International Conference on Geosynthetics, 10ICG, Berlin, Germany, September 21–25, 2014 (CD-ROM).
Correia, N.S., and Zornberg, J.G. (2016). “Mechanical Response of Flexible Pavements Enhanced with Geogrid-Reinforced Asphalt Overlays,” Geosynthetics International, June, Vol. 23, Issue 3, pp. 183-193.
Holtz, R.D., Christopher, B.R., and Berg, R.R. 1997. Geosynthetic Engineering. Bitech Publishers Ltd., Richmond, British Columbia, Canada, 452 p.
Holtz, R.D., Christopher, B.R. and Berg, R.R. 2008. Geosynthetic Design and Construction Guidelines, U.S. Department of Transportation, Federal Highway Administration, Washington D.C., Report No. FHWA-NHI-07-092, 612 p.
Koerner, R.M. (2012). Designing with Geosynthetics. Sixth Edition. Vol. 1 and 2.
Perkins, S.W., Christopher B.R., Thom N., Montestruque G., Korkiala-Tanttu L., Want, A. (2010). “Geosynthetics in Pavement Reinforcement Applications.” Proceedings of the 9th International Conference on Geosynthetics, Guarujá, Brazil, Vol. 1, pp. 165–192.
Zornberg, J.G. and Gupta, R. (2010). “Geosynthetics in Pavements: North American Contributions.” Theme Speaker Lecture, 9th International Conference on Geosynthetics, 9ICG, Guarujá, Brazil, May 23–27, Vol. 1, pp. 379-400.
Zornberg, J.G., and Thompson, N. (2010). Guide for the Application and Selection of Geotextiles, Center for Transportation Research (CTR), Document 0-5812-P1, Austin, Texas, November, 16 p.
Zornberg, J.G., Azevedo, M., Sikkema, M., and Odgers, B. (2016). “Geosynthetics with Enhanced Lateral Drainage Capabilities in Roadway Systems.” Transportation Geotechnics (in press).